top of page
Sound to Energy Device

Sound to Energy Device: Image
My sophomore year spring, my roommate and I comprised the engineering team for a Duke Bass Connections design project. The motivation of the project was to create a device that can convert the vibrations from high volume sound waves to electrical energy through piezoelectric crystals. Following the development of the product, an economic, environmental, and social benefit analysis was conducted to defend the feasibility of the technology.

Sound to Energy Device: Image
Seeing as this was a novel concept for two young engineering undergrads, the team went through great trial and error until we landed on the final design. For example, I had an idea for a cylindrical apparatus to be aimed at the source of the sound, with a low stiffness spring + mass system at the bottom. The mass would strike the piezoelectric crystal, which would be suspended by a cantilever beam on the side of the cylinder.
This iteration did not reach implementation because the spring’s displacement did not provide sufficient pressure to the piezo crystal nor a sufficiently continuous oscillation to generate useful and measurable AC current to the circuit.

Sound to Energy Device: Image
The first component of this device is the trapezoidal prism at which the sound would be directed. This was assembled using 4 cut out pieces of 6061 aluminum alloy sheet metal, held together using industrial aluminum tape for structural integrity. I elected to use 6061 aluminum because of its highly elastic property, making it susceptible to intense vibrations under the effect of loud noise.
The prism geometry was selected because the amplitude of the waves would reflect inside the device as they traveled closer to the vertex, change path and be directed nearly perpendicular to the walls, and create stronger impacts and thus greater vibrations. This is similar to the phenomenon of placing a music-playing device in a bowl and having the sensation of a louder volume because the sound is reflected back toward your ears instead of away.
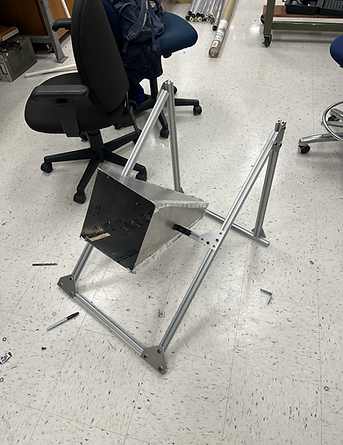
Sound to Energy Device: Image
At this point, it became apparent that the sound levels necessary to create sufficient motion of the device would most likely need to be produced from heavy machinery or an industrial plant. The team brainstormed potential areas that could work as a “target market” for the product and using a decision matrix decided on Duke University’s on-campus chiller plant, a building that continuously cools water throughout the day—and it does so quite noisily.
I tracked the decibel level of the plant using a XRCLIE-1359 decibel meter sound level reader at a set distance, about 20 meters away from the compressor (the loudest unit in the refrigeration cycle) at 5 different times, intervelled an hour between each trial. I measured a consistent 94 dB throughout the day, which for reference is about the level of noise one would experience next to a passing train in a New York subway. Thus, the chiller plant was chosen because of its ease of access, continuous sound production, and high decibel level.
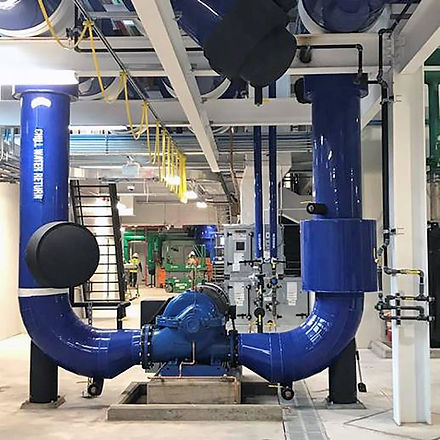
Sound to Energy Device: Image
Piezoelectric crystals are elements that produce a current under the application of continuous or repetitive pressure from the polarization of the two plates surrounding the piezomaterial. This technology has applications ranging from uses in microphones and cigarette lighters all the way to sensors for microelectronic medical devices.
One of the most common types of piezomaterials is lead zirconate titanate, the one used in this project. This perovskite—a family of semiconductors that is one of the most researched and promising rising technologies in photovoltaic solar cells—has a special property where its valence band can essentially be excited by the kinetic energy of mechanical stress. This excitement can have the electrons overcome the material’s small band gap and fill the valence band.

Sound to Energy Device: Image
The electricity generated from the piezos was AC because of the inherent electrical properties of the semiconductor. We understood that the efficiency of the device would be too low to be economically feasible or even environmentally justifiable, considering the carbon-intensive industrial process required to create the piezo crystals. We found the optimal frequency of sound to output the highest voltage by having a speaker play 10 different frequencies from 90-180 Hz at 94 dB and attaching a voltmeter at both sides of the wired arms of a single piezo unit. Through this testing, it was determined that 160 Hz produced 0.04 mV, the highest of the trials.
Next, to demonstrate proof of concept, the team originally planned on directing the electrical current to an LED. A load of a red LED only requires 10-20 mA, however it needs about 2 volts of voltage. Thus, even if all 18 piezo crystals were arranged in series (to sum the voltages) with a 94 dB source at 160 Hz (the determined ideal conditions for maximum power), it could only reach 0.72 mV. This is thousands of factors below the required 2 volts of an LED, so proof of concept would have to be demonstrated in a different way.
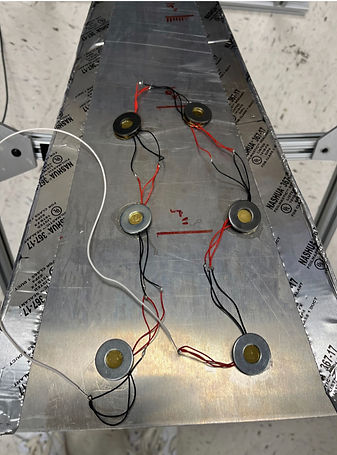
Sound to Energy Device: Image
To achieve this, we would use an oscilloscope to show the real-time voltage of the circuit after converting from AC to DC using a diode bridge rectifier. This voltage would be measured across the capacitor. It should be noted in a typical RC circuit that the voltage drop across the capacitor decreases over time, however due to the low input of voltage from the piezos in combination with the intentionally high capacitance (1000 µF for high energy storage according to U=0.5*C*V^2) of the capacitor, I decided that this dropoff would be negligible.
It should also be noted that although there is no physical resistor present, the internal resistances of the wires and diodes are sufficient to label this as an RC circuit. The idea is that the current would be directed to the capacitor, which over a long period of time would become fully charged so that it could be discharged (by flipping the switch to its second pin) through the LED with sufficient voltage.
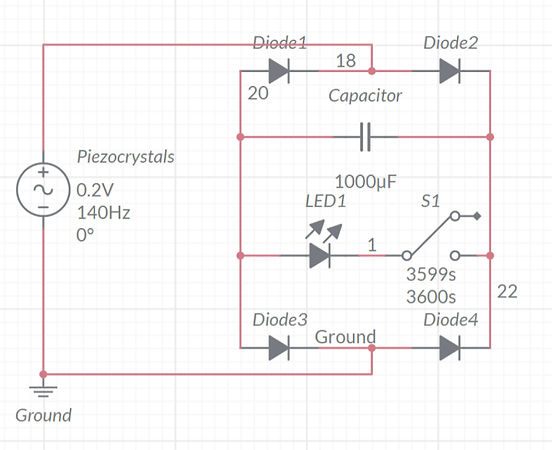
Sound to Energy Device: Image
Since the electricity sources would no longer be directed towards an LED, the piezo units could now be arranged to maximize total power instead of voltage. To accomplish this, the crystals were stacked in sets of 3, connected in parallel to sum the currents, with washers placed on top as additional mass to increase the total pressure. The 6 sets of 3 stacks were connected in parallel to sum the voltages.
With this final configuration, the oscilloscope had constant voltage over time, demonstrating the AC had successfully been converted to DC. The final value achieved was approximately 0.8 mV, just above the ideal value predicted with all units in series but was higher because of additional pressure achieved from the washers.

Sound to Energy Device: Image
There are further steps that could be taken to increase the efficiency of this sound-to-energy device:
1. Testing to determine the highest density of vibrations on the surface of the cone
2. Experimenting with different geometries of funnel based on wave reflection properties
3. Adding additional piezo crystals and finding data that would analyze the number of units and voltage output to try and see if there exists a law of diminishing returns from less vibrational effects due to more piezo units
4. Different configurations and combinations of piezo stacking and washers to accomplish useful work instead of just proof of concept
Sound to Energy Device: Image
Sound to Energy Device: Files
bottom of page